Not long ago it seemed as if reducing greenhouse gas emissions would be enough to save the world from climate change. Replace fossil-fuel power plants with clean energy sources, make cars and buildings more efficient, switch to LED lights, eat less meat, and so on. Slashing emissions and boosting renewables looked like the answer even to the Intergovernmental Panel on Climate Change as recently as 2005. But the strategy has not worked out as planned. Global emissions have gone up instead of down. It now appears that even cutting annual net emissions worldwide to zero by 2050 will not be enough.
To prevent economic and environmental devastation, climate experts maintain that we will now also have to achieve negative emissions. Doing so means removing billions of tons of carbon dioxide from the atmosphere every year. That is like saying we can no longer put out the garbage—and we need to steadily take back the garbage we put out in the past.
Negative emissions on a massive scale have become “a bio-physical requirement” to meeting climate change goals, according to a 2018 study led by Jan C. Minx of Germany’s Mercator Research Institute on Global Commons and Climate Change. Figuring out how to get there is a matter of “immediate urgency,” he and his co-authors warn in Environmental Research Letters, if the world hopes to limit warming to 1.5 degrees Celsius. Almost every nation on the planet subscribed to that target—with a fallback of “well under” two degrees C—as part of the 2016 Paris Agreement on climate change. Warming is currently about one degree above preindustrial levels. But it is increasing at 0.2 degree C per decade. In a special report in October 2018, the Intergovernmental Panel on Climate Change warned that we have just 12 years to act if we hope to avoid slipping past 1.5 degrees C, the level regarded by most scientists as the furthest we can go if we hope to preserve life more or less as we know it.
On supporting science journalism
If you're enjoying this article, consider supporting our award-winning journalism by subscribing. By purchasing a subscription you are helping to ensure the future of impactful stories about the discoveries and ideas shaping our world today.
Staying under that threshold mandates a specific “carbon budget,” an overall amount of carbon dioxide we can add to the atmosphere without pushing warming beyond that temperature. At today’s emissions—about 40 billion to 50 billion tons a year—“there may be only five years’ worth of CO2 emissions left” in the 1.5-degree scenario, Minx and his co-authors say. (For the remainder of this article, we use “tons” to mean “metric tons.”) After that, every additional ton would require an equal withdrawal. His group estimates the world will need to remove 150 billion to more than one trillion tons of CO2 from the atmosphere by 2100—roughly two billion to 16 billion tons a year, starting in 2050, with the number increasing significantly later in the century.
To do that, Minx and his team note, we will have to start building “several hundred” carbon capture and storage installations a year beginning in 2030, just 11 years from now. That could mean deploying big machines to pull carbon dioxide from the air or developing bioenergy power plants that burn trees—grown in continuous rotation—in a facility that captures emissions and sends them deep underground for permanent burial. Low-tech options would include replanting cut forests or expanding existing ones, improving farm and pasture soils so they hold more carbon, and crushing and spreading certain kinds of rock that soak up CO2.
Most of the higher-tech carbon capture methods are still in the early stages of development, however. They require enormous investment at considerable risk of failure and entail major side effects, including competition for land that is already being used to feed people or provide habitat for wildlife.
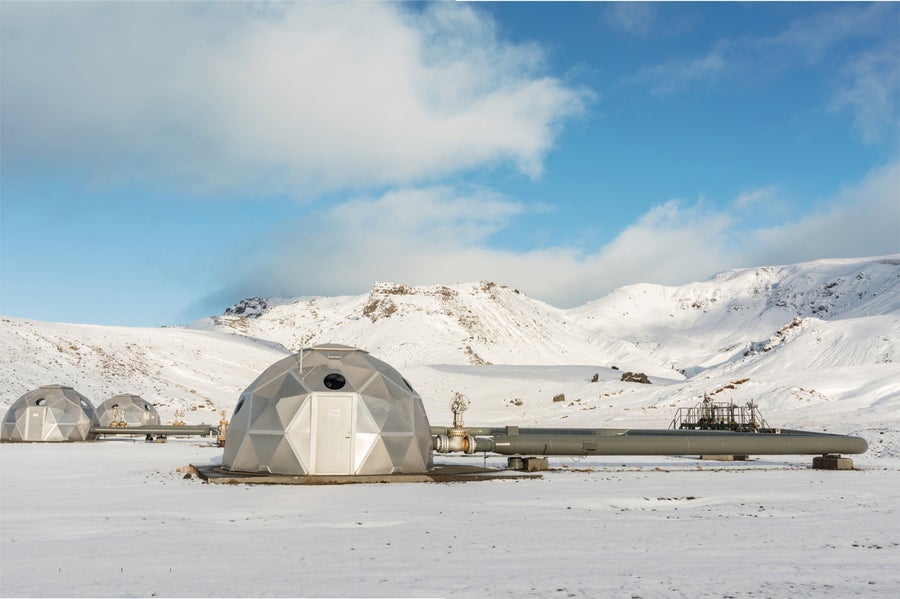
Climeworks machine extracts carbon dioxide from the atmosphere. Credit: Liz Tormes
And yet pursuing carbon capture on a massive scale appears to be our only option. When University of Washington statistician Adrian E. Raftery and his co-authors of a 2017 study in Nature Climate Change looked at current trends—not including negative emissions technologies—they found that we are on track to reach 3.2 degrees C of warming by the end of the century, with a range from two to 4.9 degrees C. In a subsequent study in the Proceedings of the National Academy of Sciences USA, Texas A&M climate scientist Yangyang Xu and a colleague categorized warming greater than three degrees C as “catastrophic” and greater than five degrees C as posing “existential threats to a majority of the [human] population.”
.png?w=900)
An injector inside a nearby dome sends the carbon dioxide more than 700 meters underground. Credit: Liz Tormes
So let us assume that one trillion tons of negative emissions in this century—an average of 20 billion tons a year from 2050 to 2100—is necessary. What share of that pie could each method account for and at what cost? Given competition among methods for certain resources, such as land, what is the best mix to pursue? And can we muster the political will to pursue negative emissions—while drastically cutting our current carbon dioxide output?
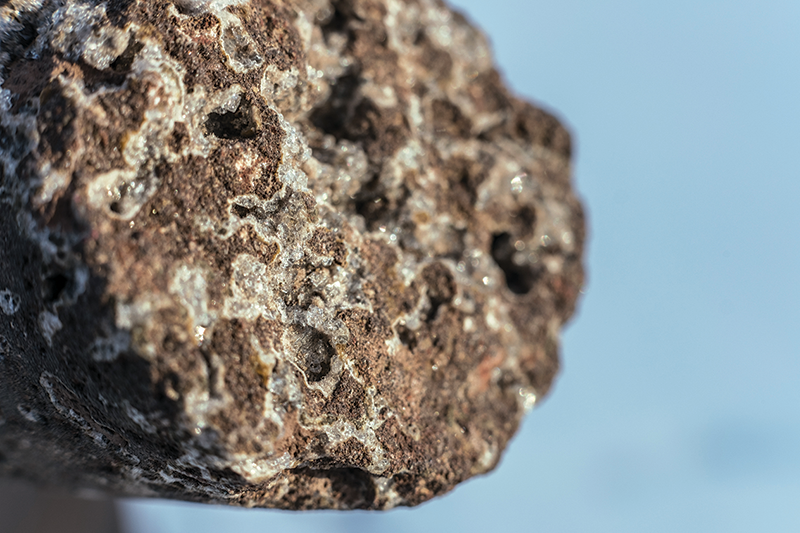
The gas reacts with basalt bedrock to form carbonate, visible as white streaks in a core sample from the basalt. Credit: Liz Tormes
Clean the wind
On a hardened lava field of boulders and moss in the foothills just outside Reykjavík, Iceland, a machine the size of a one-car garage pulls air through a chemical filter that extracts carbon dioxide. It is powered by waste heat from the geothermal power plant next door, and it pumps the captured carbon dioxide more than 700 meters underground, where the gas reacts with basalt rock and becomes solid mineral. Climeworks, a Swiss start-up, calls the operation the first direct air capture and storage plant in the world. It sequesters a modest 50 tons of carbon dioxide a year.
Direct air capture and storage may be the most straightforward path to negative emissions: banks of fans would harvest CO2 from the sky and bury it. Scientific scenarios project that this technology could remove 10 billion to 15 billion tons of carbon dioxide a year by the end of the century; a few experts think 35 billion or 40 billion tons may be possible. This is such a tantalizing prospect that many climate scientists worry it could pose a moral hazard: people might think they can delay fossil-fuel reductions now in the hope of technological salvation later.
The most thorough review of removal methods—another 2018 study in Environmental Research Letters—takes a more sober view. Sabine Fuss of Mercator and her colleagues examined costs, side effects, environmental sustainability and other factors to project the carbon sequestration potential for seven major removal methods. Fuss and her co-authors put that potential for direct air capture in 2050 at only 500 million to five billion tons a year—adding up to 25 billion to 250 billion tons this century—at a cost of $100 to $300 per ton. For perspective, our cars each typically emit 4.6 tons of carbon dioxide a year.
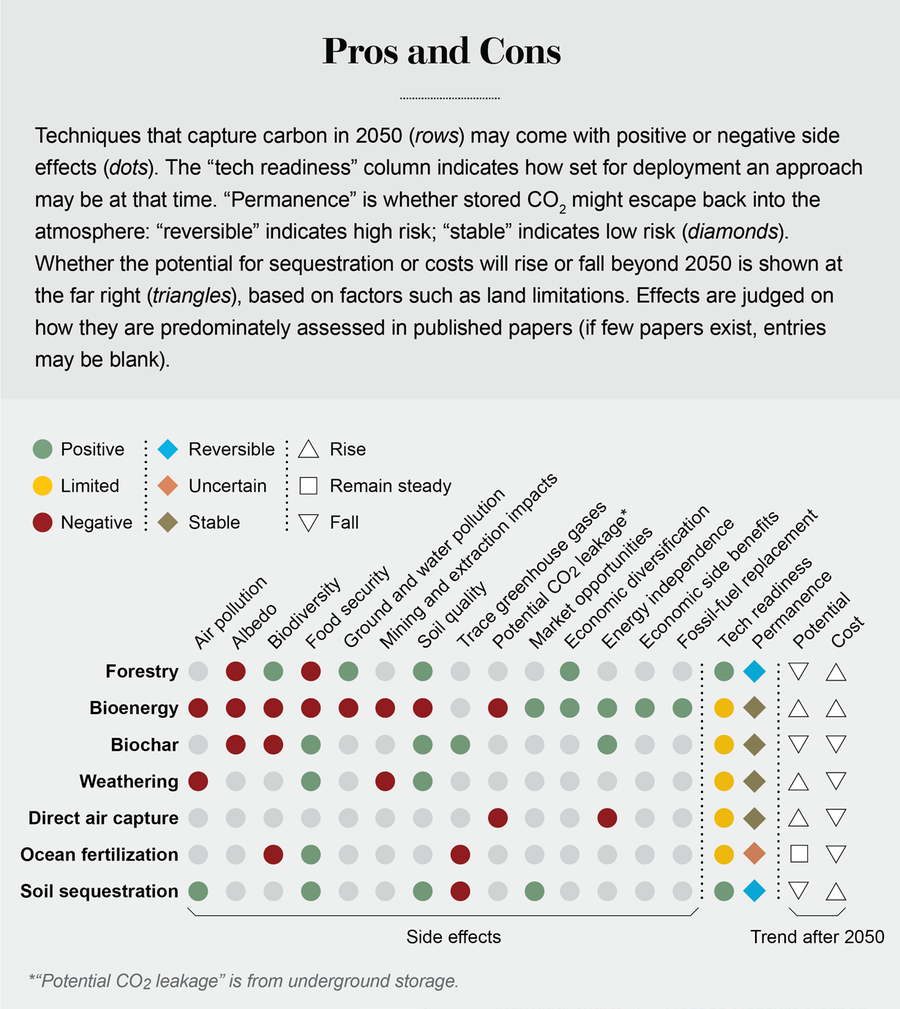
Credit: Pitch Interactive; Source: “Negative Emissions—Part 2: Costs, Potentials and Side Effects,” by Sabine Fuss et al., in Environmental Research Letters, Vol. 13, No. 6, Article No. 063002; June 2018
Indeed, air capture “is not a silver bullet,” says James S. Mulligan of the World Resources Institute’s Food, Forests, and Water Program. “It’s not a shiny object. It’s kind of a cruddy object. But we need it.” Some researchers claim they could get the cost below $100 per ton. Yet if the time line for doing that at scale is anything like the 60-plus years it took solar power to move from satellites in the 1950s to broad marketplace penetration today, Minx says “it may be too late.”
Direct air capture also consumes enormous amounts of energy. Removing a million tons of carbon dioxide a year would require a 300- to 500-megawatt power plant, according to Jennifer Wilcox, a chemical engineering professor at Worcester Polytechnic Institute. If that were a coal-fired plant, it would create more emissions than it would remove. If power came from solar or wind farms, it would cover a lot of land that might already be in demand for farming or nature. And of course, a million tons would barely make a dent in the target of 20 billion tons a year.
Constructing such plants now might be essential to developing the know-how for building them on a much larger, more efficient scale later in the century. “But if you went out and built 20 million tons of direct air capture today, that would be the wrong thing to do with your money,” says Roger Aines, chief scientist of Lawrence Livermore National Laboratory’s energy program. “It would take a lot of solar and wind power, and if you had that much solar and wind power, the best thing to do would be to put it on the grid and turn off a coal plant.” Preventing new emissions is still the overwhelming priority.
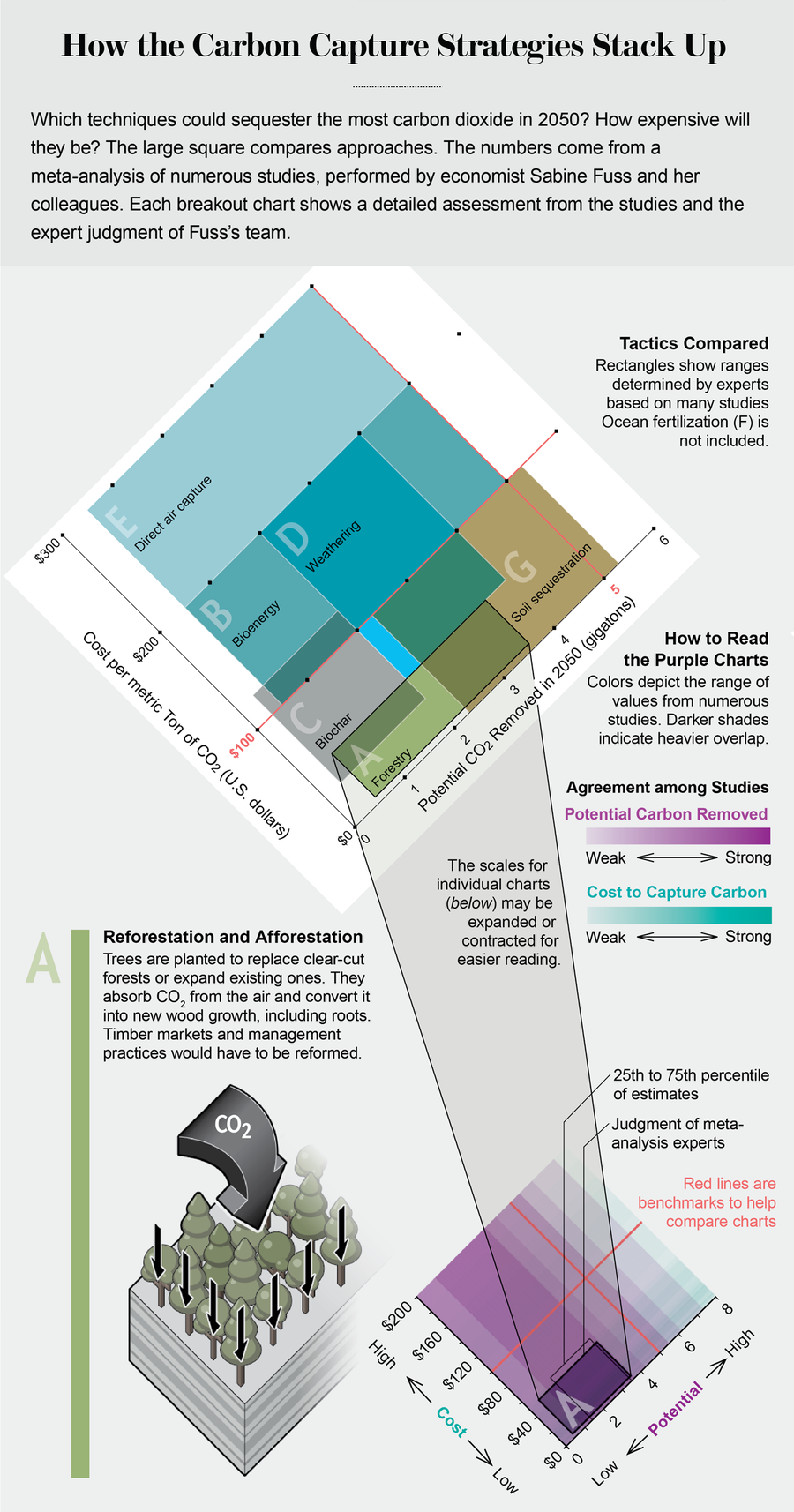
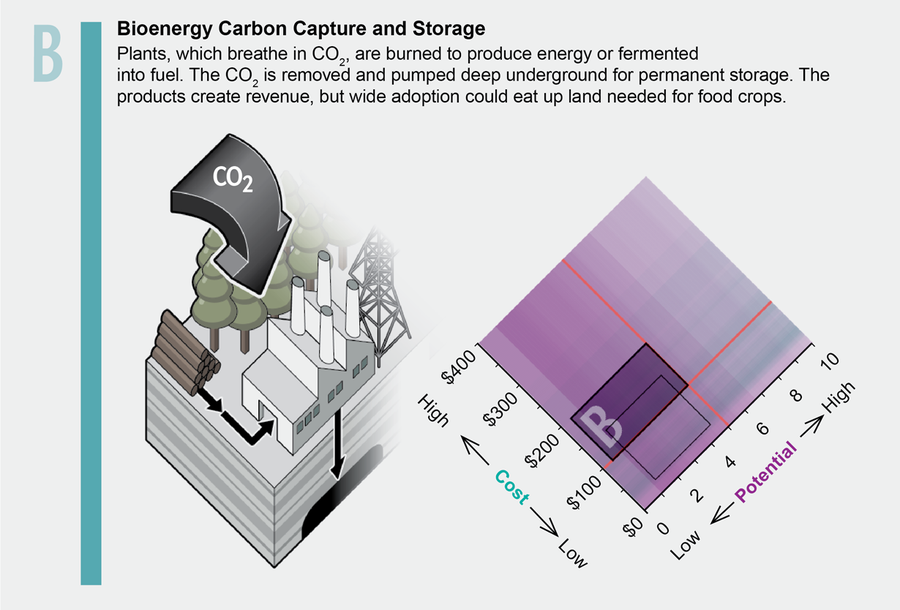
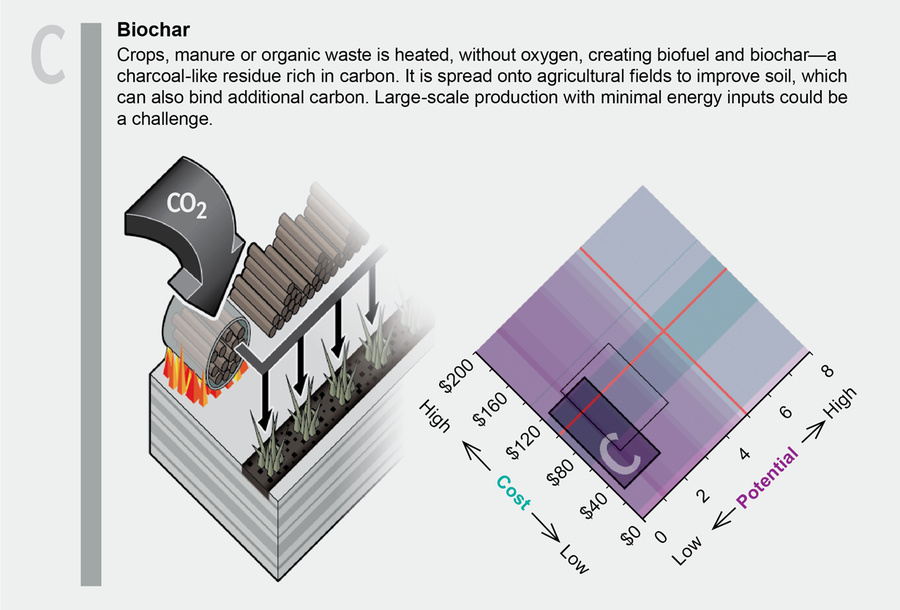
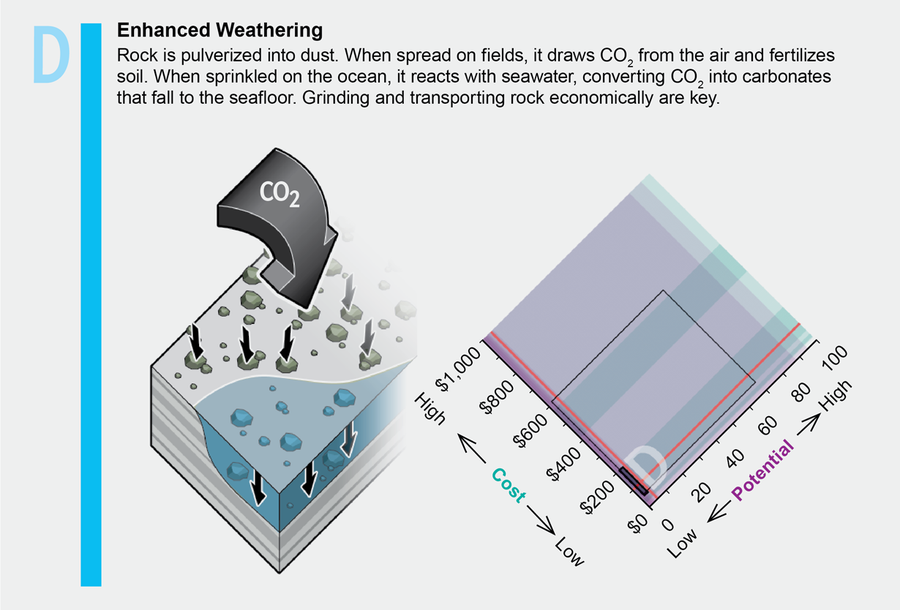
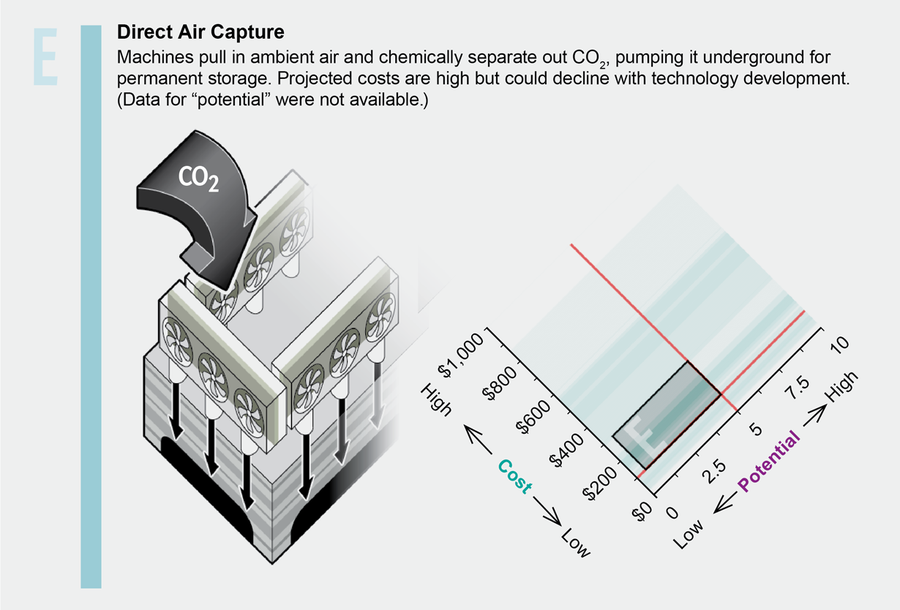
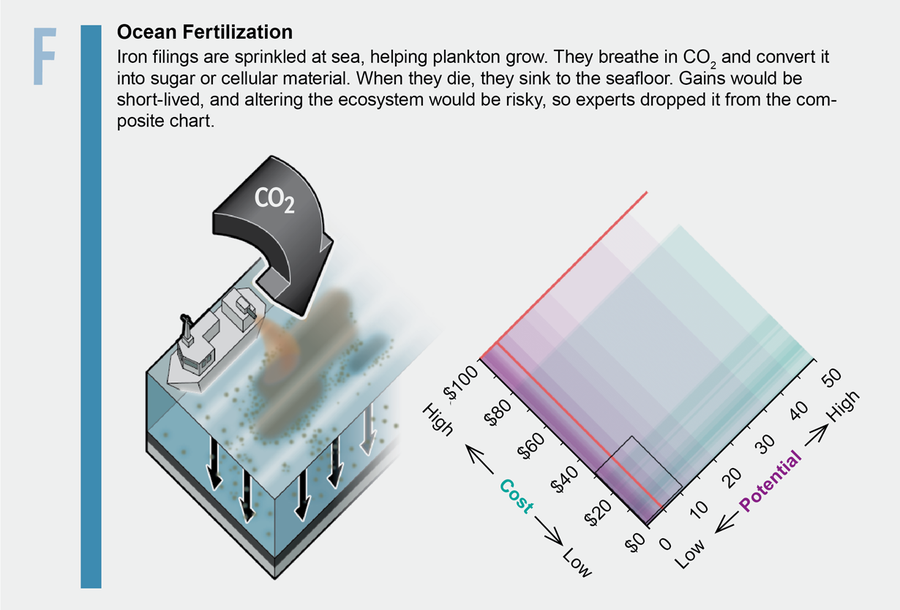
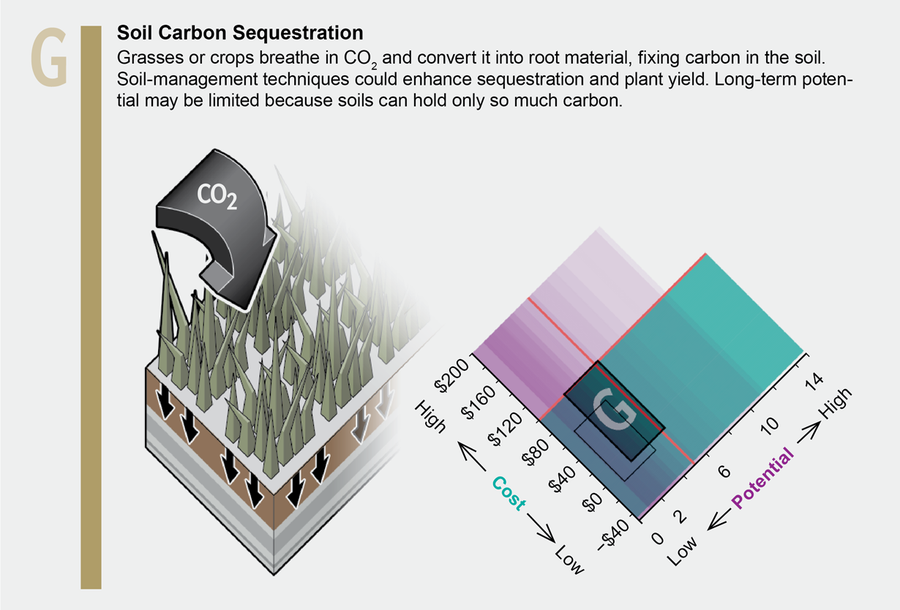
Credit: Pitch Interactive (graphic), Ben Gilliland (illustration); Source: “Negative Emissions—Part 2: Costs, Potentials and Side Effects,” by Sabine Fuss et al., in Environmental Research Letters, Vol. 13, No. 6, Article No. 063002; June 2018
Portfolio of fixes
The Fuss study does not simply add up the potential of the seven carbon capture methods, because some of them compete for the same resources. For example, too much reforestation would take away land needed to grow the fuel for bioenergy power plants, and too much bioenergy might compete with direct air capture for underground carbon sequestration. Climate scientists say we need to optimize a portfolio of methods.
One way to start building this portfolio, says Pete Smith, a professor of soils and global change at the University of Aberdeen in Scotland, is by scaling up “stuff we already know how to do. We know how to plant trees. We know how to restore peatlands, basically by raising the water table,” so the peat captures carbon dioxide instead of emitting it. “We know how to improve soil carbon content.... Incentivizing those sorts of things is [relatively] easy and could be done immediately. It would get us some of the way there.”
Consider reforestation. Tragically, the world’s tropical forests have become a source, rather than a sink, for carbon dioxide emissions, as trees are cut down and burned or as ravaged forests degrade. Getting forests back into negative emissions territory would first require major reforms in an international timber market that is heavily influenced by illegal trade. Beyond that, the obvious place for reforestation is land that had been clear-cut for farming or grazing but was abandoned as unproductive. Restoring five million square kilometers of such land could sequester 3.7 billion tons of carbon dioxide a year if adequate funding were available, according to a 2015 study in Nature Climate Change led by Richard Houghton of the Woods Hole Research Center.
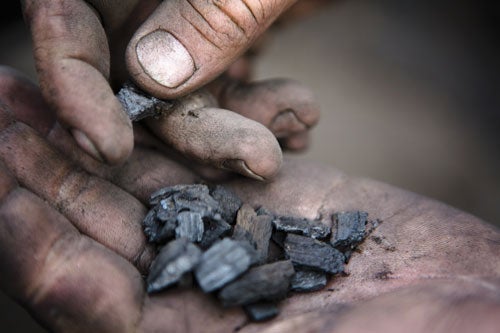
BIOCHAR fertilizer was made by heating chicken waste and wood chips that would otherwise emit carbon dioxide as they decay. Credit: Jeff Hutchins Getty Images
Turning all livestock grazing lands that used to be forested back into forests again could create as much as 10 billion tons of negative emissions a year, according to Bronson W. Griscom, senior director of forest carbon science at the Nature Conservancy and lead author of a study on “natural climate solutions” in PNAS. That is a sizable portion of the annual carbon dioxide recovery needed annually. But the move would require a global shift away from meat eating, the opposite of the current trend.
Fuss and her co-authors foresee more modest potential. Trees live and die, meaning they will store carbon now but give it up again later this century or the next. The amount of carbon dioxide sequestered will also likely decline as forests mature, growing more slowly. Wildfires, deforestation and climate change raise the risks. Even so, forest expansion could provide a critical stopgap while direct air capture or other technologies are scaling up. Fuss puts the potential somewhere between 500 million and 3.6 billion tons of carbon dioxide removal a year by midcentury. That could take 25 billion to 180 billion tons off our target of one trillion tons this century, at a cost of $5 to $50 per ton.
Better management could raise the gains. Griscom notes, for example, that tree plantation managers in the southeastern U.S. knowingly harvest loblolly pine trees several years before their optimal yield. Allowing them to sell carbon credits to cover the extra years of growth could delay harvesting to the optimal age, adding on more timber and more carbon storage.
Likewise, growing nitrogen-fixing plants in pastures and moving to a smarter system of pasture rotation could make grazing more productive while improving carbon storage in soils. Fuss conservatively estimates that improvements in soil sequestration can yield up to 5.3 billion tons a year—265 billion tons this century—at $0 to $100 per ton.
That would be in addition to biochar. In this form of carbon removal, a specialized furnace applies heat in the absence of oxygen to biomass, turning it into a form of charcoal and generating useful by-products such as bio-oil or synthetic gas. When the charcoal is applied to farm fields, it binds carbon in the soil and can improve crop yields. But no one has yet attempted to deploy biochar on a large scale. Fuss and her co-authors consider it a plausible source of 300 million to two billion tons of annual carbon dioxide removal, at $90 to $120 per ton. That is 15 billion to 100 billion tons in this century.
Another land-based approach is called bioenergy with carbon capture and storage, or BECCS. Early plans from many countries for meeting their Paris commitments depend on it, yet it is deeply controversial. A power plant burns wood, agricultural wastes or other biomass such as switchgrass. These sources take carbon dioxide out of the atmosphere as they grow or accumulate. Combustion releases it again, and the power plant recaptures it from the smokestack, sending it down into deep geologic formations for permanent storage. But reforestation for biofuel production at the scale suggested by some proponents could eat up much of the world’s arable land, threatening food production and nature conservation, as well as carbon dioxide removal by other methods such as reforestation or soil sequestration. Pulling emissions from the smokestack also sharply reduces power plant efficiency, at least with current technologies. Hence, Fuss puts the sustainable yield from BECCS at just two billion tons a year, well below the forecast by other researchers, at a cost of $100 to $200 per ton. Fuss’s estimate would account for 100 billion tons of negative emissions by 2100.
That leaves two other carbon capture methods now under consideration. Enhanced weathering exploits a natural process: carbon dioxide in the air converts into carbonate when exposed to certain kinds of crushed rock. The question is whether researchers can find a way to grind the right rocks into powdered form economically, to speed up the natural process. Fuss puts the potential at two billion to four billion tons a year, at $50 to $200 per ton. Her team concludes that ocean fertilization—sprinkling iron or other nutrients into the ocean to stimulate growth of algae and other plankton, which take up carbon dioxide—would be too inefficient and short-lived to justify potential ill effects on ecosystems. It is “not a viable negative emissions strategy,” they write.
Profit rather than cost
Where does the accounting leave us? The ranges in the Fuss study add up to as little as 150 billion tons or a bit more than one trillion tons by 2100. The latter number might sound as if it solves our problem. But we cannot just add up the numbers because of conflicts between methods. What we can do, Fuss says, is manage the portfolio to take advantage of beneficial overlaps. Enhanced weathering, for instance, could be deployed on the same land being used to grow biomass for BECCS.
What all the approaches need, scientists argue, is massive investment in research and development. “This is going to be a long, hard battle,” says Lawrence Livermore’s Aines. But governments have been reluctant to foot the bill for negative emissions technologies because of ideological resistance to “picking winners” and because some past investments have been notorious failures. The U.S. Department of Energy, for instance, has spent huge sums of money on carbon capture projects intended to make “clean coal” power generation a reality. Southern Company abandoned the latest attempt in 2017, switching the Kemper County clean coal plant in Mississippi to natural gas after spending $7.5 billion.
A carbon tax would bypass picking winners by imposing a cost on emissions—a cost for putting garbage into the atmosphere. That would create a marketplace motivation both to reduce emissions now and to claw back past emissions later. The U.K. imposed such a tax, currently at about $25 per ton, primarily on fossil-fuel power plants, which cut coal emissions in half just from 2015 to 2016. Most governments shy away, however, seeing a tax as too drastic for economies built on fossil fuels.
With few exceptions, corporations have also been reluctant to invest in CO2-removal technologies because, until recently, they saw no marketplace. To them, fixing the climate is a public benefit, not something from which they can earn a profit. But that may be changing because of a surprisingly bipartisan package of tax incentives approved by the U.S. Congress in early 2018. The so-called 45Q legislation significantly increases the tax credits companies can claim over the next 12 years, not just for capturing carbon dioxide and sequestering it underground—at as much as $50 per ton in tax credits—but for using CO2 in a variety of ways.
The most controversial use is “enhanced oil recovery.” An oil company purchases carbon dioxide, transports it by pipeline and injects it into depleted oil wells, pushing out extra oil it could not extract by conventional means. A climate change solution that entails producing more fossil fuels may sound Orwellian, and some environmental critics have attacked 45Q as just another fossil-fuel subsidy program in disguise. But enhanced oil recovery appears to reduce current emissions because the captured carbon dioxide, typically from natural gas or ethanol refineries, gets sequestered underground. Some environmentalists, such as Kurt Waltzer of the Clean Air Task Force, argue that turning carbon capture into an energy technology, rather than an emissions technology, is the first step toward broad commercial adoption of carbon dioxide removal. It turns recaptured CO2 into a product to be bought or sold rather than simply a cost to be endured. That could be the key to eventual negative emissions.
Time to start
Could a portfolio of carbon capture methods, taxes and markets get us to the goal of one trillion tons by 2100? The record-breaking temperatures of 2018 and 2019 may have been a turning point. The American West was on fire. People on four continents experienced severe heat waves. In Japan, thousands of heatstroke victims went to the hospital in a single week. Even the Arctic experienced a major heat wave, and 3,500 square miles of Amazon forest went up in flames, darkening the skies over São Paolo. Climate scientists shook off cautious language and warned, in PNAS, that further warming risks tipping the planet into a “Hothouse Earth ... likely to be uncontrollable and dangerous to many.” In case that message was not strong enough, senior author Hans Joachim Schellnhuber, director emeritus of the Potsdam Institute for Climate Impact Research in Germany, told reporters that the cascading effects could lead to a world capable of supporting just one billion human beings, down from 7.5 billion today.
For some political leaders even now, climate change still seems shrouded in uncertainty, despite the overwhelming evidence that it is our grim present and grimmer future. The disconcerting thing about negative emissions technologies is that so much can seem uncertain even for the scientists themselves. “Everyone is talking about how it depends on what the substrate is, what part of the world you’re in, what the rainfall is like there and what the temperature is,” says University of Virginia ecologist Stephanie Roe, speaking about soil carbon enhancement. Likewise, carbon sequestration in forests began to look far less certain as apocalyptic wildfires burned across Australia.
Researchers also get caught up in arguing about whether any of the carbon-removal methods, much less all of them, can scale up to billions of tons annually. “There’s maybe a bit too much focus in this debate about what’s the eventual scale,” says Brendan Jordan of the Great Plains Institute in Minneapolis. “I fear that it paralyzes us, and we really can’t afford paralysis.” That is, we need to start to achieve negative emissions despite uncertainties because they are trivial compared to a world in which the climate change game of musical chairs stops, and there’s no room for 6.5 billion people to sit down.